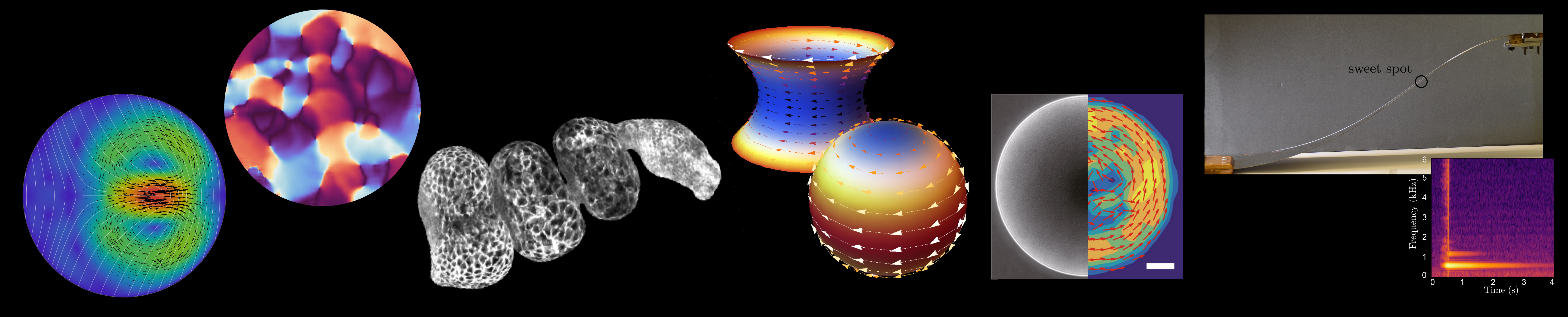
Biological Physics
Living systems, from subcellular to super-organismal scales, harness the interconversion and transport of energy, mass, momentum, etc., to accomplish remarkable biological functions. Understanding how their physico-chemical and material nature constrains and enables biological processes is of key importance, not only to understand life in the natural world, but also to engineer new biomimetic properties in synthetic materials. But how do we address the heirarchical and multiscale complexity of living matter? How can we incorporate sensing, feedback, and adaptation in a variable environment? Can we make sense of the diversity of form and function in the animal and vegetal world? To make progress, we adopt a mixed approach that combines the study of specific model systems with minimal theoretical descriptions and comparative analyses. A common theme of interest is asking how physical forces, flows, and form dictate function in physiology, its breakdown in pathology, and its origin in development, all contextualized (when possible) in an ecological or evolutionary framework.
Physiology
One of the most basic signatures of life is autonomous motion and locomotion. How can we understand its range and limits across the tree of life? Plants use the movement of water through soft tissues to generate rapid nastic motions, but in animals, muscles are the primary effector of behavior and motion. We have recently shown that intracellular flow of water within a soft, fluid filled muscle fiber constrains its ultimate rate of contraction. Such active hydraulic effects are particularly relevant in ultrafast muscle driven movements such as in insect flight. As muscle is active, its mechanical response is also found to be nonreciprocal (‘odd’) – an unusual property violating the symmetry between response and perturbation. Nonreciprocal mechanics allows muscle to function as an active elastic engine that can generate power using spatial strain cycles, a mechanism that is potentially employed in physiology. Other ongoing projects include ultrafast contractions of single cells, the onset of peristalsis, and the response of gut bacteria to inflammation.
Morphogenesis
How do organs and organisms shape themselves? Understanding the mechanisms of morphogenesis is one of the major open challenges in biology. It is also a natural realm where patterns of chemical signalling (morphogens), gene expression, growth, and active mechanics all interplay to generate complex, functional morphologies. Tissues use changes in cell number, position (in animals), shape, and size to effect large-scale morphogenetic events. How cellular and sub-cellular processes are coordinated in space and time to generate organ scale geometry remains a formidable open question. In a developing fruit fly embryo, we have shown how genetically controlled calcium patterns can drive muscular contractions that fold the epithelial tissue (endoderm) forming the midgut. Integrating molecular details with tissue-scale active mechanics and large-scale environmental perturbations to characterize organ growth, morphology, and variability is a current open problem we are pursuing in plants. A very different, but related problem is understanding the dynamics and morphology of growing multicellular colonies. In this context, how growing populations of microorganisms compete and evolve in fluid flow (e.g., in the ocean, due to swimming etc.) is also of interest.
Relevant publications
- Active muscular hydraulics, S. Shankar, L. Mahadevan, 2022 [bioRxiv:2022.02.20.481216] (in-review)
- Visceral organ morphogenesis via calcium-patterned muscle contractions, N. P. Mitchell, D. J. Cislo, S. Shankar, Y. Lin, B. I. Shraiman, S. J. Streichan, eLife 11:e77355 (2022) [DOI] [PDF] [biorXiv]
- Spatial population genetics with fluid flow, R. Benzi, D. R. Nelson, S. Shankar, F. Toschi, X. Zhu, Rep. Prog. Phys. 85 096601 (2022) [DOI] [PDF] [arXiv]
- Shape and size changes of adherent elastic epithelia, B. Loewe, F. Serafin, S. Shankar, M. J. Bowick, M. C. Marchetti, Soft Matter 16, 5282-5293 (2020) [DOI] [PDF] [arXiv]